To consider an application for financing, fill out the form and send it to us by e-mail along with the project brief, or contact our experts
- Solar photovoltaic power plant construction
- Innovative materials for solar PV power stations
- Thin-film solar technology
- Advanced solar PV modules
- New areas of application for solar PV power stations
- Technologies and operating principles of solar photovoltaic power plants
- Production and principle of operation of photocells
- Charge controllers for solar photovoltaic power plants
- Choosing inverters for solar power plants
- Communication systems for solar photovoltaic power plants
- Overvoltage protection of solar power plants
- Construction of solar PV power plants: economic feasibility and cost
- Construction of solar power plants for industrial enterprises
- EPC contracting in solar energy
Among the reasons for investors' interest in renewable energy sources are growing concerns about climate change, the effects of air pollution on health, the issue of energy security and affordability, as well as fluctuations in hydrocarbon prices.
Currently, the total installed capacity of PV power stations in the world exceeds 600 GW, excluding concentrating solar systems.
It is the second largest renewable energy source after wind farms.
Since the late 2010s, this technology has been a leader in the pace of construction of new power plants.
These figures are twice as much as that of wind farms.
They are even higher than those of fossil fuel plants and nuclear power plants together.
In 2020 alone, the installed PV capacity is planned to be increased by 140 GW.
According to experts from the International Renewable Energy Agency (IRENA), the development of solar energy is driven by cost savings, technological advances and the creation of the necessary associations to support the sector.
Solar photovoltaic power plant construction
Given the availability of economic and technological resources, significant market potential and competitiveness, it is expected that photovoltaic technologies will continue to lead in the field of renewable energy in most regions of the world over the next decade.According to IRENA forecasts, the number of new solar photovoltaic stations can increase 5 times over the next 10 years, reaching a total capacity of 2840 GW by 2030 and 8500 GW by 2050.
This means that the installed PV capacity in 2050 will be 18 times more than in 2018. According to European experts, in 2050, 60% of the installed capacity will come from large-scale photovoltaic installations, and the remaining 40% from in-roof PV systems.
Although large-scale power plants will dominate in 2050, we expect faster growth in distributed PV systems, which are supported by government policies and incentives.
Asia dominates the global solar energy market today, accounting for more than half of the world's new photovoltaic capacities.
In 2019, China added over 30 GW of installed capacity, while the European Union added 16 GW and the United States 13.3 GW.
Construction of new solar photovoltaic power stations in 2019:
Country | New installed capacity, GW |
People's Republic of China | 30,1 |
European Union (total) | 16,0 |
United States of America | 13,3 |
India | 9,9 |
Japan | 7,0 |
Vietnam | 4,8 |
Spain (EU) | 4,4 |
Germany (EU) | 3,9 |
Australia | 3,7 |
Ukraine | 3,5 |
South Korea | 3,1 |
Asian countries, led by China, are currently leading in the production of photovoltaic energy.
Europe is in second place and North America in third.
Projections show that Asia will continue to lead in installed PV capacity with a share of about 65% of total capacity in 2030. The most significant growth is expected in China, where the installed PV capacity will exceed 1,400 GW in 2030.
North America will take second place with 430 GW by 2030, with 90% of the facilities being built in the United States. Europe will occupy third place with an installed capacity of about 300 GW.
In 2050, Asia will still dominate with almost half of the installed photovoltaic power in the world. According to the estimates, this figure will be 4,800 GW, of which 2,800 GW will be concentrated in China. By then, Chinese solar power will show CAGR of about 9%.
North America will retain its second position with an installed photovoltaic capacity of 1720 GW.
The United States will continue to dominate the region.
Europe will retain third place with a total installed PV capacity of 890 GW in 2050. About 22% of European PV installations will be concentrated in Germany.
At the same time, market growth is likely to shift to other, less saturated markets. In the future, the rapid development of solar energy is expected in South America and Africa.
The future growth of solar energy depends largely on a balanced energy policy and a reduction in the cost of PV technology. The ways to achieve this are to use cheaper materials for solar cells, reduce the cost of manufacturing equipment and increase its efficiency.
Innovative materials for solar PV power stations
Today, we are witnessing continuous progress in research and development for both existing and new photovoltaic technologies in order to further reduce costs and increase productivity.Crystalline silicon (c-Si) cells are the first generation of photovoltaic cells, accounting for 95% of world production.
Due to the use of the common materials, silicon c-Si panels are more affordable and efficient than other solutions.
Over the past few decades, solar cells have improved significantly in terms of efficiency and power output. The average efficiency in 2006 was 13.2% for polycrystalline and 14.7% for single-crystal photovoltaic panels. Since then, this indicator has been growing steadily, reaching 18% and 19%, respectively. It is expected that this positive trend will continue until at least 2030.
Currently, the strong position of c-Si in the market makes it difficult to develop other technologies. However, despite the high level of efficiency of this first-generation photovoltaic technology, there are many opportunities for improvement.
Among them:
• Lower cost of c-Si modules for higher profits.
• Reduction of metal inclusions and prevention of defects.
• Limiting environmental impact through waste reduction.
• Obtaining finer elements due to improved material properties.
PERC technology (Passivated Emitter and Rear Cell) uses an advanced silicon cell architecture. The structure of the PERC elements is slightly different from the structure of typical single-crystal cell.
The key improvement here is the integration of the surface with the passivated layer, which increases the efficiency of the cell.
The passivated layer achieves this by the recombination of electrons, increasing the absorption of light, and providing a higher internal reflectivity.
The increase in the efficiency of solar cells due to the implementation of the PERC architecture for single-crystal cells is about 0.8-1%, while the growth for polycrystalline cells is slightly lower, from 0.4 to 0.8%. PERC technology has only recently entered the global market, but is quickly becoming the new industry standard for single-crystal photocells.
Tandem solar cells are a set of individual elements located one above the other. Each of these layers converts light with a specific wavelength, allowing residual light to be absorbed and converted into electricity by the lower element.
New technologies in solar energy include several options for tandem cells, which can be grouped by the materials used (organic, inorganic, hybrid), as well as by the type of connection used.
In practice, tandem cells have been used to design the world's most efficient solar cells, which can convert up to 50% of the energy of sunlight into electrical energy. Unfortunately, these devices use very expensive materials and production processes, so it is difficult to bring them to market.
Thin-film solar technology
Thin-film solar cells are also called second-generation photovoltaic panels.The semiconductor materials used in the production of thin-film elements have a thickness of only a few microns.
These elements include two main varieties, including silicon-based elements (amorphous and micromorphic a-Si / c-Si) and non-silicon elements (perovskites, cadmium telluride and copper-iridium-gallium selenide, CIGS).
Thin-film solar cells can be cheaper to manufacture and easily commercialized, but their performance is still low.
Currently, most solar cells are made of silicon. But one of the areas that our engineers are focusing on is the development of new materials.
One of the most promising is perovskite, a rare mineral with a very high ability to absorb light. The first perovskite devices in 2009 converted only 3.8% of the energy of sunlight, but their efficiency is rapidly increasing.
Since crystals are very easy to make in the laboratory, their production is increasing. In 2018, the efficiency of perovskite solar cells has already reached 24.2%, which is close to the efficiency of laboratory silicon cells (26.7%).
Unfortunately, perovskite photocells still face a number of serious problems that hinder their distribution. One of them is low stability. Since perovskite crystals dissolve readily, they cannot be used in humid environments. Such elements require moisture protection by coating them with a layer of alumina or other materials.
Another problem for scientists is that the high efficiency of small perovskite solar cells is difficult to reproduce on larger systems.
If these barriers can be overcome, technology will change the economy of solar energy. Perovskite elements are much cheaper to manufacture and do not require such difficult high-temperature production conditions as silicon devices.
CIGS solar cells are also relatively highly efficient compared to crystalline silicon cells. But large-scale industrial production of CIGS elements is difficult in the short term due to the high cost of indium, complex stoichiometry and multi-stage production.
Cadmium telluride elements currently have an efficiency of more than 21%, similar to that of CIGS.
This material is characterized by rather high absorption and low energy loss.
CdTe cells are produced using low-temperature processes, which makes their production flexible and affordable. CdTe is currently the market leader among the major thin-film technologies in the solar industry.
Advanced solar PV modules
The emergence of new architectures contributes to the efficiency of solar photovoltaic power stations.The main driving force behind this change is the PERC elements (passive emitter and rear module) and their compatibility with other innovations.
In the future, the most important technological trend in the market is associated with the development of bilateral solar cells and modules. Bilateral solar PV cells have been developed for decades. It is not surprising that today this production process can be considered one of the most reliable and well-established.
Bilateral elements generate electricity not only from sunlight entering the face, but also from reflected light entering the back.
Today, China is a development leader, the world's largest manufacturer and end-market for bilateral photovoltaic modules.
Demand for these elements is growing worldwide. In particular, bilateral solar cells are actively used in PV installations for public utilities in Brazil, the United States, and the UK.
According to experts at the Fraunhofer Institute for Solar Energy Systems, almost every PERC solar cell manufacturer is also working on bilateral solar PV cells.
The use of such PV modules provides an increase in efficiency of approximately 5–20% with the same cell area. Despite the growing market and the obvious advantages of bilateral solar cells, the new technology must overcome some problems, such as the lack of an international testing standard, as well as problems with modeling energy production.
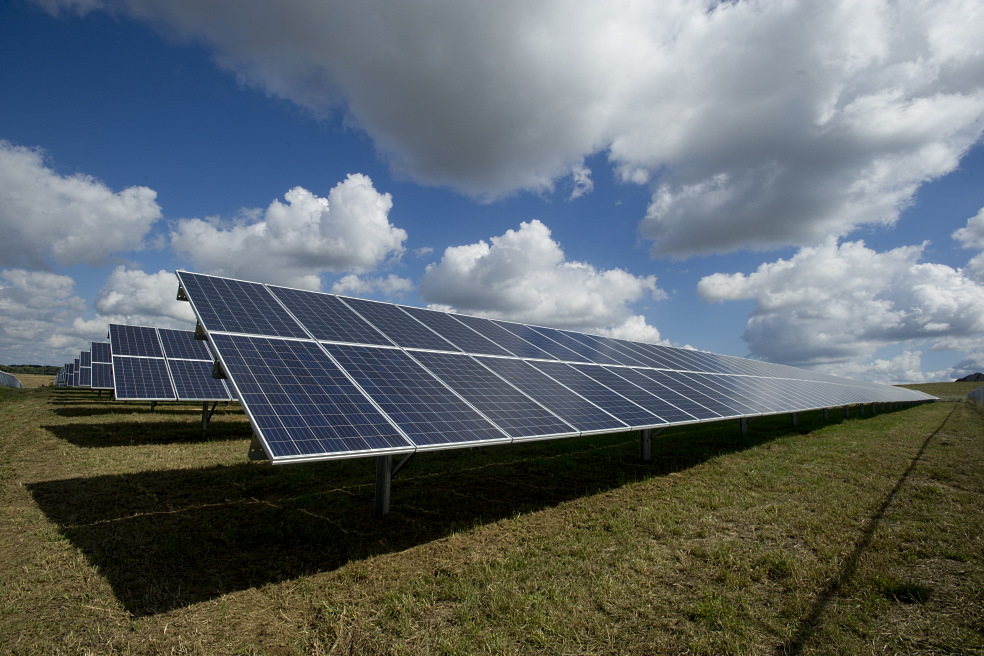
One option for double-sided modules is a glass-glass solar panel.
These are devices whose photocells are located between glass panels.
They are widely used for utilities and are considered the optimal solution for harsh and inhospitable environmental conditions (such systems are more resistant to moisture).
Despite considerable experience in the production of such panels, the high cost and significant weight of the equipment inhibits market growth. As of 2020, their market share reached 10%, but within 10 years it is expected to grow to 40%.
Semi-cells are photocells cut in half, which are produced using advanced laser machines. The development of this technology is facilitated by the simplicity of the process and minimal changes in the operation of laser machines.
Semi-cells increase the efficiency and durability of PV modules, offering an immediate increase in efficiency. Thanks to PERC integration, the semi-cell technology brings the efficiency of traditional systems to 18% or more.
Silicon solar cells are metallized in thin stripes on the side and back surfaces.
These buses are required to conduct the current generated by the cell.
Older panels usually have two buses, but the industry is moving toward higher efficiency, and the number of buses currently reaches 3, 4, or even more. A larger number of buses is associated with higher efficiency of the PV modules due to reduced losses due to internal resistance.
New areas of application for solar PV power stations
Floating photovoltaic plants are a growing market with potential for rapid growth.According to a World Bank report, at the end of 2018, the installed capacity of floating systems reached 1.1 GW.
The demand for floating photovoltaic plants is growing, especially in island and other land-limited countries where the cost of placing offshore platforms is lower than onshore solar PV stations.
Floating solar power plants are particularly suitable for Asia, where there is a shortage of land, but there are significant hydrological resources with a ready-made infrastructure. Europe also has enormous potential for floating PV systems, especially in the Netherlands and France.
However, like any new technology, floating solar power plants experience numerous technical difficulties that must be overcome. In particular, this is the lack of reliable anchor systems and problems with navigation.
Another growing trend in the field of solar energy is the integration of solar panels in residential and commercial buildings.
This approach has several advantages.
Firstly, the building integrated PV element (BIPV) is multifunctional, as it can be adapted to almost any external surface. These devices perform passive and active functions. A key passive function is heat and sound insulation. Among other unique advantages of integrated photovoltaic systems in buildings, experts consider the possibility of regulating heating and lighting in real time.
Secondly, BIPVs are a promising cost-effective solution for investors.
They offer potential in terms of lowering the cost of roofing materials and labor.
Compared to conventional roofing materials, BIPV systems are more expensive. But taking into account the additional income from the generated electricity, these higher initial investments pay off quickly. Among the benefits of BIPV are versatility and flexibility in terms of design.
Another technology, solar parking, is also gaining popularity.
These are ground solar photovoltaic panels under which cars are parked. In addition to providing shade for vehicles, the panels generate electricity, which can be used, for example, for electric vehicle charging stations.
Finally, solar parking can provide the possibility of additional energy storage in the presence of such an integrated system. The growth of the solar parking market is determined by several factors. The difference in cost between in-roof photovoltaic systems and conventional roofing structures continues to decline, making them an attractive solution for investors.
The driving force behind this market is also utilities, which are increasingly relying on local distributed energy generation as a way to increase the reliability of the electricity network.
Solar trees are very similar to real ones, as they have leaf panels connected by metal branches. They can be considered as an addition to solar photovoltaic installations on the roofs of buildings.
As a rule, solar trees are more ergonomic compared to horizontal systems and take up almost 100 times less space to generate the same amount of energy. This makes solar trees a suitable solution for regions, cities and companies with limited space that do not have the possibility of mass construction of traditional solar PV power stations.
Today, most desalination plants run on fossil fuels, making them unsustainable in the long run.
The two most popular methods of desalination and the production of drinking water are the membrane method (reverse osmosis, nanofiltration and electrodialysis) and the thermal method (various types of distillation).
Since membrane technology does not require heating, such installations can be combined with a wind turbine or solar photovoltaic system. Due to the significant drop in prices for photovoltaic equipment and the growing demand for drinking water, experts expect mass construction of new desalination plants powered by solar energy.
Agrivoltaic uses solar panels for agriculture, including the cultivation of various crops under ground-based photovoltaic panels.
Although this concept was proposed a long time ago, until recently, little attention was paid to agrivoltaics. Today, many scientists have confirmed the benefits of its implementation, such as producing more electricity, achieving higher yields and using less water to irrigate the fields.
Photovoltaic thermal hybrid solar collectors (PV-T) combine the production of electrical and thermal energy in one system.
These include a solar panel with a cooling system in which special refrigerant (water or air) circulates around the solar cells. Here, the refrigerant is heated so that thermal energy can be used efficiently for various applications.
The cooling system of photovoltaic panels has a double advantage. It significantly increases the efficiency of electricity production and accumulates heat, using it for space heating, water heating and industrial processes.
In fact, most modern photovoltaic modules use only about 15-20% of the incoming solar energy, the rest is lost in the form of heat.
New trends in solar energy are aimed at solving this problem.
Technologies and operating principles of solar photovoltaic power plants
Today, solar photovoltaic power plants are the backbone of renewable energy.Photovoltaic cells come in a variety of types and sizes, from postage stamps to panels that span tens of square meters.
Photovoltaic cells connected together form solar modules.
The modules, in turn, can be combined and connected to form photovoltaic systems of different sizes and power outputs. The efficiency of a photovoltaic system depends on many factors, including the technologies and cell materials used, the intensity of solar radiation, etc.
In addition to photovoltaic panels, a solar power plant contains mounting structures, tracking systems, batteries and power electronics (inverter, controller and grid connection equipment).
A brief history of solar energy
Everyone knows that photovoltaic systems convert solar energy into electricity.However, few people know the interesting origin of the term "photovoltaic".
The word first appears at the end of the 18th century. It consists of two parts - "photo", derived from the Greek word "light", and "volt" in honor of the discoverer of electricity Alessandro Volta.
The photoelectric effect was first discovered by the French physicist Edmond Becquerel back in 1839. Photovoltaic systems have long been a part of our everyday life. Today, solar power plants provide consumers with one of the most affordable and cleanest forms of energy.
After the discovery of selenium photoconductivity at the end of the 19th century, a new stage in the development of solar energy sector began. In 1941, the first selenium photocell was constructed, with an efficiency of approximately 1%.
In 1955, Western Electric Company was the first company in the world to commercialize solar panels.
Since solar radiation is much higher in space, these low-efficiency cells have been of limited use to power satellites and space stations.
In 1958, the first solar-powered satellite, Vanguard 1, was launched. Today it is considered the oldest operational satellite. It was followed in 1962 by Telstar, which was the first communications satellite to be equipped with 14W photovoltaic cells. In 1971, Soviet space stations participating in the Salyut programme were equipped with this technology.
In the 1970s, driven by the oil crisis, NASA and the US Department of Energy began developing a project to power the Earth using satellites. In 1979, a satellite fleet was proposed with an installed capacity of up to 10 GW, but in 1981 the project was closed due to the high cost of manufacturing these satellites.
The first solar photovoltaic power plants were developed in the early 1980s, and most of them were built in the United States. By the 1990s, almost all developed countries began to generate electricity using this technology, among which were Japan, Spain, Germany, Italy and others.
The important role of China in the construction of solar power plants should be emphasized. By 1997, this country had surpassed the United States to become the largest solar energy producer in the world.
Today, China is also one of the world leaders in the manufacture of equipment for solar power plants.
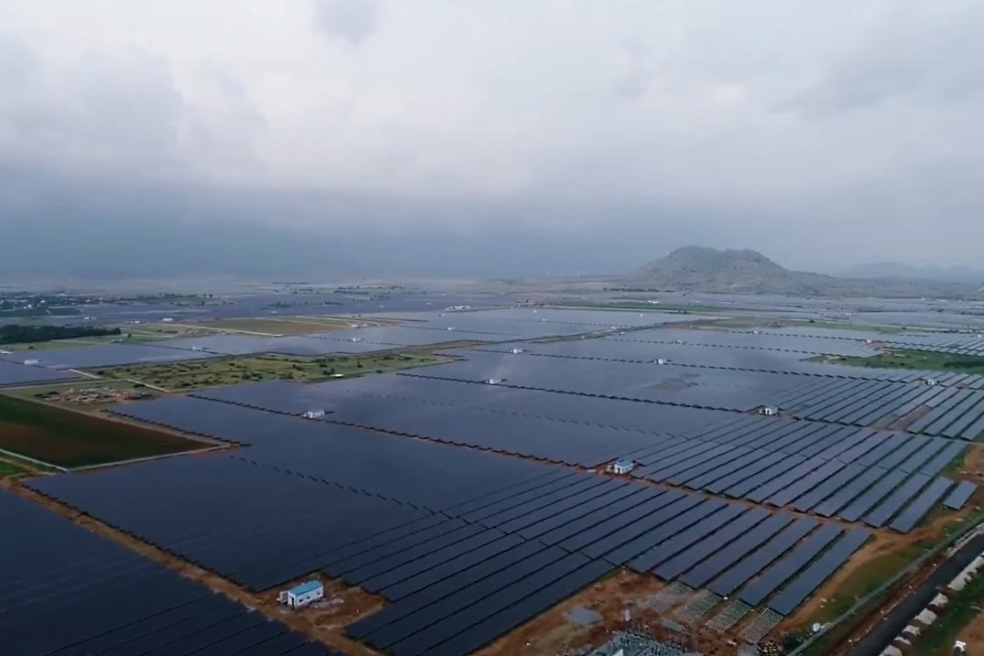
Using sunlight to generate electricity
Solar energy is vital to our planet. It determines the temperature of the Earth's surface and ensures the course of numerous biological processes.How does a photovoltaic cell convert solar energy into electricity?
To answer this question, we need to understand the properties of sunlight.
Some stars provide energy in the form of X-rays and radio signals, but the sun emits most of the energy in the form of visible light. However, visible light is only a fraction of the electromagnetic spectrum. Almost all the energy of the Sun has the wavelength from 2x10-7 to 4x10-6 meters.
Each electromagnetic wavelength has a specific frequency and energy. The shorter the wavelength, the higher the frequency and the more energy it carries. For example, red light lies at the low energy end of the visible spectrum, and violet light lies at the opposite end of the spectrum.
The same is observed in the invisible part of the electromagnetic spectrum: ultraviolet light is high energy, and infrared light is low energy. Therefore, infrared light, which we perceive as heat, carries less energy than visible light.
Photocells react differently to waves of different lengths. For example, crystalline silicon uses the visible spectrum and part of the infrared spectrum. But the amount of energy in the infrared spectrum is too little to generate electricity.
However, light containing too much energy also cannot be effectively used by photovoltaic cells to generate electricity.
The main reason is that some of this energy is converted to heat.
How powerful is solar radiation?
The sun constantly emits a colossal amount of energy.The planet receives a tiny fraction of this energy.
For every square meter of the outer layer of the Earth's atmosphere, there is an average of 1,360 watts of solar energy. The atmosphere absorbs and reflects some of this radiation, including most of the X-rays and ultraviolet rays. However, the amount of solar energy that reaches the earth's surface every hour exceeds the total amount of energy that humanity uses in a whole year.
How much energy does light lose as it travels from the upper atmosphere to the Earth's surface?
These losses depend on the thickness of the atmosphere through which the sunbeam must pass. The energy that reaches sea level at noon in temperate latitudes with clear skies reaches 1000 W / m².
As the sun moves lower and lower in the sky, the sunbeam travels through an increasing layer of air, losing more energy. Since the Sun stays at its zenith for a short period of time, the available energy during the day is significantly less than 1000 W / m².
Features of direct and diffused light
As noted, Earth's atmosphere and clouds absorb, reflect, and scatter some of the sunlight. However, the fraction of solar energy that reaches the Earth's surface is sufficient to generate solar power. It should be borne in mind that part of the solar radiation is direct, and the other is scattered.The distinction between the two types of sunlight is extremely important. Some solar power plants can use both types, however photovoltaic systems can effectively work only with direct sunlight.
Direct light includes radiation emanating directly from the Sun, which is not reflected from clouds, dust, the earth's surface, or other objects. Experts talk about "perpendicular radiation", meaning the portion of light that comes directly from the sun and hits the surface of a photovoltaic module at an angle of 90 degrees.
Diffuse light means the sun's rays that are reflected by clouds, dust, the earth's surface, or other objects. Obviously, reflected light is much more difficult to reach the module than direct sunlight. Scattered light cannot be focused by the concentrator optics.
Total solar radiation is defined as the total amount of solar energy falling on a horizontal surface. It consists of two components, direct (perpendicular) and diffuse light. It should be borne in mind that scattered and direct sunlight has different spectra.
The structure of a solar photovoltaic power plant
Overall, a solar power plant is a simple and practical system for generating affordable electricity in places where it is expensive to use the electrical grid.In addition to photovoltaic modules, the construction of solar power plants includes cabling, installation and configuration of auxiliary electrical equipment, battery connection and much more.
Solar PV modules
Solar PV modules are a set of elements responsible for capturing solar radiation and converting it into electrical energy. It is the most important and most expensive part of any photovoltaic system.Controllers
The intensity of solar radiation can vary significantly depending on the weather. On some days, solar cells operate at minimum power, while on others, excess sunlight overloads the power plant.The controller is a small electronic device that monitors the amount of electricity coming from the panels, preventing overloading the system due to excessive solar radiation.
Inverters
Photovoltaic cells generate constant electrical current, but the vast majority of electrical appliances require alternating current.An inverter is a device that converts direct current from solar panels into a type of energy that can be used and transmitted over long distances.
Batteries
Batteries are responsible for storing electrical energy when the radiation source is not available.More efficient and reliable electrical energy storage systems have been developed recently. Among them are liquid air energy storage systems (LAES), introduced a couple of years ago by the British innovation company Highview Power.
Types of solar photovoltaic power plants
Today, solar power plants can be seen in the most isolated places on Earth and in the heart of megacities.There are different types of systems, including:
• Autonomous photovoltaic solar systems.
• Solar photovoltaic power plants connected to the grid.
• Solar photovoltaic power plants with a backup generator.
• Hybrid PV solar systems, etc.
Autonomous photovoltaic systems
Such power plants generate electricity independently of the power grid. In some cases, off-grid solar photovoltaic systems are considered a more profitable solution than alternative grid expansion. They are especially suitable for places with high ecological requirements and remote areas such as national parks.In rural areas, small off-grid solar photovoltaic power plants power home lights, electrical security systems, and water pumps. Most of them are equipped with rechargeable batteries.
Solar PV power plants connected to the grid
For maximum efficiency, most PV systems are connected to a central power grid.During hours when the energy consumption is less than the production capacity of the system, part of the generated electricity is supplied to the grid. Conversely, the system draws energy from the grid when the current production capacity of the solar cells is insufficient.
This type of solar photovoltaic system eliminates the need for costly storage batteries, although connecting to the mains can be difficult. According to the current legislation of a number of European countries, electric companies are obliged to buy solar energy at preferential prices.
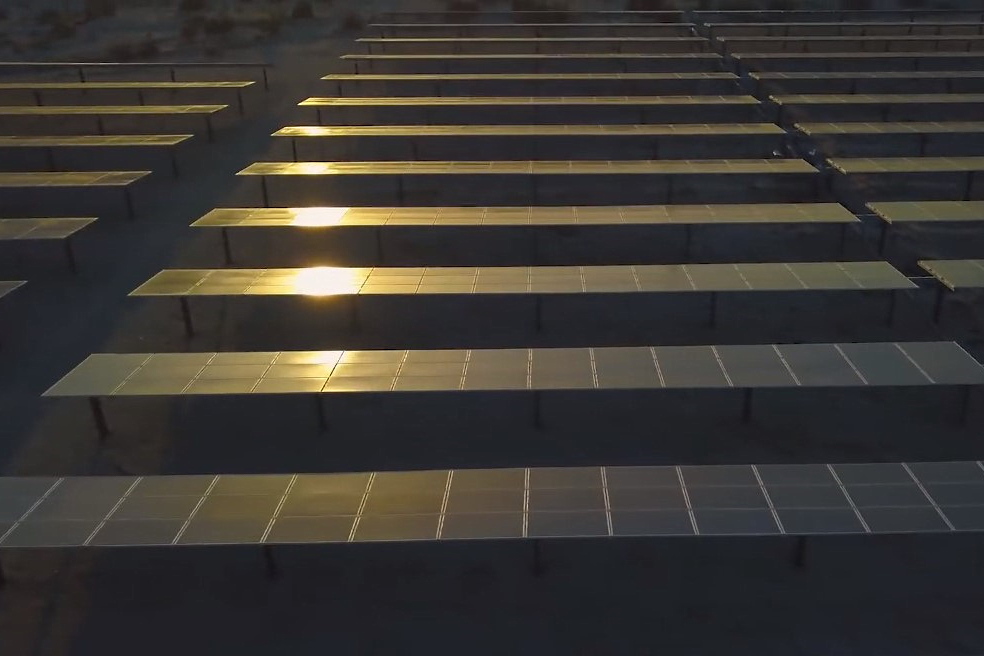
Hybrid solar photovoltaic systems
Hybrid systems meet the energy needs of consumers by combining several solutions to store excess solar energy.In addition to photovoltaic modules, they include diesel generators, wind generators, small hydroelectric power plants and other sources of electricity depending on location and available energy resources.
Hybrid solar PV systems are considered the optimal solution for powering remote consumers such as communication stations, military facilities and holiday villages.
They are widely used all over the world to power seismic monitoring stations located, for example, in the middle of the ocean.
The effectiveness of hybrid solutions depends on how accurate the preliminary analysis of a specific application is, including energy consumption, available energy resources and their cost of use. This will enable the design of a hybrid system that best suits the energy needs of customers.
Production and principle of operation of photocells
Solar photovoltaic cells, which convert solar radiation into electricity, are predominantly made from semiconductor materials.One of the most widely used materials for solar power sector is crystalline silicon (c-Si).
At the moment, this is the most affordable material from which photocells are made. It is used in several forms: monocrystalline silicon, polycrystalline silicon, sheet silicon, and thin-layer silicon.
Popular technologies for the production of crystalline silicon include the Czochralski (CZ) method, the floating zone (FZ) method, and other technologies — casting, extrusion, or drawing.
Removing impurities and defects in silicon is of particular importance for the efficient operation of photovoltaic cells. This is done using techniques such as surface passivation (surface treatment with hydrogen) and gas absorption (chemical heat treatment that causes diffusion of silicon impurities).
Amorphous silicon thin-film solar cells
Since around the 2000s, thin-film photocells have taken an increasing share of the market. These elements consist of layers of semiconducting materials a few micrometers thick, deposited on an inexpensive base such as glass, flexible plastic, or stainless steel.Semiconductor materials deposited as thin films contain amorphous silicon (a-Si), copper-indium diselenide (CIS) and cadmium telluride (CdTe). Amorphous silicon has no crystalline structure, and its properties gradually deteriorate under the influence of light due to the Stebler-Wronsky effect. The described effect can be reduced by hydrogen passivation.
Since the amount of semiconductor material required for thin-film coatings is significantly less than that of traditional photovoltaic cells, their manufacturing costs are significantly lower compared to older types of crystalline silicon cells.
Other materials for the production of photocells
Chemical elements of groups III and V in the periodic table are highly effective in natural and concentrated sunlight.Monocrystalline cells of this type are usually made from gallium arsenide (GaAs).
A feature of gallium arsenide is that its doping with indium, phosphorus and aluminum creates semiconductors characterized by a high conversion efficiency of sunlight with different characteristics.
The drive to improve the efficiency of solar photovoltaic power plants has led to the development of highly efficient multi-pn junction cells.
They are packs of individual solar cells stacked on top of each other to maximize the capture and conversion of solar energy.
The top layer of the photocell converts sunlight, which contains the most energy. The layer freely transfers the remaining solar energy to other layers, which absorb and transform it. Gallium arsenide and its alloys, as well as amorphous silicon, copper-indium diselenide, and gallium-indium phosphide are widely used for the production of such high-performance solar cells.
Although dual pn junction solar cells have been developed, most research has focused on 3-4 pn junction cells using materials such as germanium.
The goal of this work is to capture the radiation in the lower layer as much as possible.
Innovative photovoltaic cells
In some products, instead of semiconductor materials, solar cells are made of a titanium dioxide layer treated with light-sensitive paint.Since titanium dioxide is relatively inexpensive, such photovoltaic cells can significantly reduce project costs.
Other technologies are based on the use of polymer materials.
Photoelectrochemical cell technology, which generates hydrogen from water in the presence of sunlight, also has significant potential.
Industrial production of ready-made solar panels
The widespread use of photovoltaic cells for generating electricity is associated with the need for mass production of blocks of several solar cells (panels).Optimizing mass production offers great opportunities in terms of increasing the competitiveness of solar PV plants.
The technical problems are mainly related to the removal of impurities contained in the semiconductor material. Impurities have a major impact on the efficiency of photocells, and removal techniques can be quite expensive.
The key stages of the production process are surface passivation of materials and application of special anti-reflective coatings.
Placing photovoltaic modules in a protective housing is another important step in the manufacturing process.
Charge controllers for solar photovoltaic power plants
The main function of the solar charge controller is to protect the battery, monitor the charging process and prevent it from discharging at night.The simplest and cheapest charge controller is a conventional two-position circuit breaker (on / off).
When the battery power drops, the controller enables solar panel charging to the maximum level at any voltage value, regardless of battery requirements. When the sun goes down, the device disconnects the solar panels from the battery.
Solar modules are characterized by constantly changing power output due to the changing position of the sun during the day.
Their performance also depends on the temperature and cloud cover at a particular time. A controller with on / off positions must operate at any solar panel output voltage and load fluctuations. Typically, the maximum energy consumption does not coincide with the periods when solar cells generate maximum power.
When charging the controller, the state of charge of the battery is critical. Most batteries can be charged to a very high level when they are completely discharged.
On the other hand, even a fully charged battery requires minimal charging.
Types of solar charge controllers
The higher the amperage, the faster the battery will charge.This is especially important when sunlight is only available for a few hours a day.
But if you do not reduce the current shortly before reaching a full charge, the battery can overheat and start emitting gases, which will lead to irreversible damage.
One way to avoid this problem when charging with constant current regardless of the charge level is to use a modern Pulse Width Modulated (PWM) charge controller. Although it also has two on / off states, the on and off pulses at the output of the cell are adjusted depending on the battery level.
When the battery voltage drops, the duration of the ON mode increases. When the battery level reaches its maximum, the OFF mode becomes longer. Many advanced solar charge controllers use PWM to regulate the charging voltage as this limits the sulfation of batteries.
While a PWM charge controller significantly improves battery charging, it does not provide a combination of current and voltage when solar power is used at its maximum efficiency.
This technology does not provide the optimum voltage for charging the battery.
Among the solar PV controllers currently available on the market, the most efficient and most expensive is the Maximum Power Point Monitoring (MPPT) device. This smart controller adjusts voltage and current and analyzes the effect of input parameters on output power.
The monitoring system looks for a kink in the I-V curve of each solar panel, because at this point, the specific voltage and current values provide maximum power.
Since the MPPT charge controller continuously adjusts the voltage and current at the output of the solar panel to maximize power output, it must also regulate the voltage and current supplied to the batteries.
The goal is to make the charging process as efficient and short as possible.
The MPPT controller can effectively use the additional generated voltage that exceeds the required value to charge the battery — it is lost when using a cheaper solar charge controller.
MPPT controllers can be used on solar panels with a higher output voltage by charging an additional low voltage battery. The introduction of this technology, in turn, allows you to save on electrical equipment. In particular, install smaller and cheaper fuses, power disconnectors and electrical cables with small cross-sections.
Most MPPT controllers can handle quite large discrepancies between panel output voltages and battery voltages, making them a truly versatile option for various solar photovoltaic systems.
Redirecting excess solar energy
Some solar charge controllers have an additional feature to redirect excess energy to another consumer.This is a common practice for large solar power plants that are not connected to the grid at certain times of the year.
Consider a situation where solar cells provide more energy than batteries can store. Instead of wasting energy for the rest of the day, the controller detects the battery level and then redirects the excess energy generated by the sun to other useful purposes.
This could be, for example, a heating element installed at the bottom of a water tank.
The controller will then use excess energy for industrial heating, significantly impacting the economic performance of the PV system, including the return on investment.
Temperature compensation
All chemical reactions are temperature dependent, and this is completely true for the energy conversion processes that take place in batteries.The ideal operating temperature for batteries with immersion electrodes is about 25 ° C.
This is the temperature at which the manufacturer's specifications for these batteries are verified.
To maintain the best possible battery performance and extend battery life, the charging voltage should be increased in situations where the ambient temperature is below that specified. Conversely, the voltage decreases when the ambient temperature is higher than nominal.
Batteries require different charging rates depending on the temperature of the plates inside.
Some controllers have an additional built-in temperature sensor that measures the ambient temperature and adjusts the charging voltage accordingly.
It is assumed that the battery will be installed next to the controller at the same temperature. However, this is not always the case. Temperature differences can be significant, especially when the charge controller is mounted high on an insulated wall and the batteries are close to the ground.
More expensive charge controllers are equipped with an external temperature sensor, the signal of which is fed to a separate input.
Since the sensor is attached to the battery, the temperature readings are very accurate, which results in the most accurate regulation of the charging process.
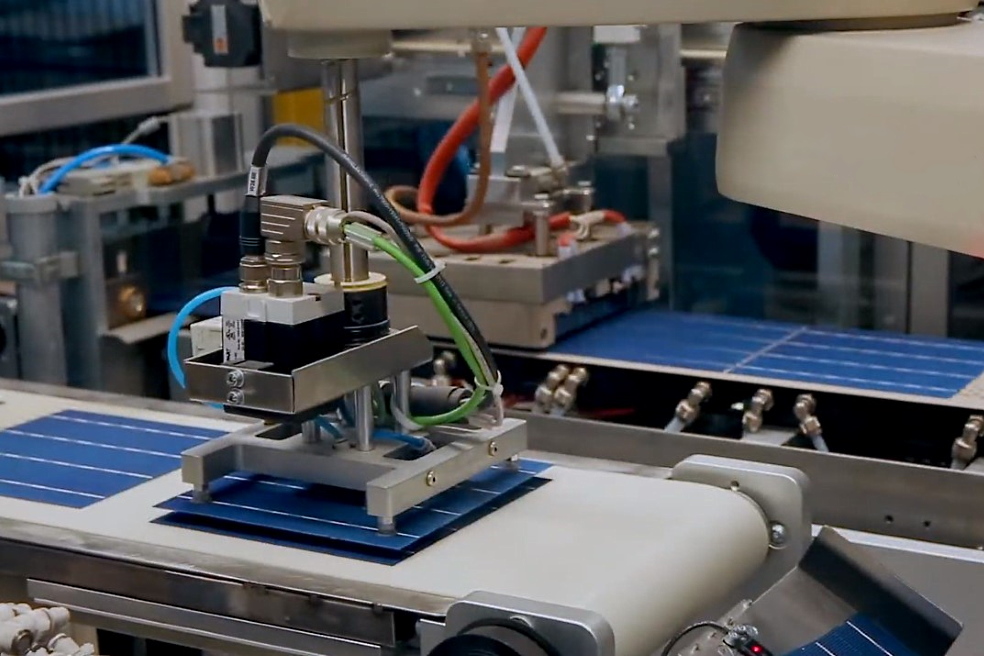
Photovoltaic system visualization
Most manufacturers of charge controllers for solar photovoltaic power plants offer sophisticated devices with built-in or remote digital display.All relevant information can be displayed here – from the output voltage of solar panels and batteries to charging currents, program setpoints and alarms from an automated system.
While this seems unnecessary for small solar power plants, displaying such data can be invaluable in large industrial facilities where parameters must be monitored and adjusted in real time.
Choosing inverters for solar power plants
The correct phase, frequency and voltage of the three-phase AC output are critical for fast and accurate synchronization of the grid-connected PV system.Converting DC to AC requires the connection of a special electronic component, an inverter.
Depending on the configuration and the components used, inverters are divided into different categories. This classification is based on factors such as the number of power handling stages (single and multi-stage), configurations with and without a transformer, and the type of switching.
Inverters are usually classified into two main types depending on the switching mode – slave inverters and stand-alone inverters. The former depend on the parameters of the network, and the impulse devices work based on the polarity or direction of the current.
The latter have full control over the pulse switching process.
Single-stage and multi-stage inverters
The single-stage inverter has various functions such as controlling the currents in the grid, boosting the voltage and controlling the maximum power.In a single-stage inverter, using a transformer increases the weight of the equipment and results in a 2% reduction in peak efficiency. On the other hand, the use of a transformerless converter makes them more efficient and lighter. They are increasingly replacing the previous type of inverters.
An inverter with more than one power stage is called multistage. In inverters of this type, the last stage performs the function of converting DC to AC, while the starting (and intermediate) stages provide voltage amplification and, in some cases, the function of galvanic isolation.
Inverters without a transformer are an economical solution and offer higher efficiency. However, to solve the problem of direct current injection into them, additional elements are required.
Communication systems for solar photovoltaic power plants
The importance of communication and control systems for large solar power plants in the near future will increase due to two factors.First, there is the potential for cost savings as a result of the introduction of the latest technological innovations.
Secondly, this is a steady increase in requirements for the safety and compatibility of equipment with the power grid.
It is expected that solar photovoltaic power plants will become one of the most cost-effective tools for generating renewable electricity. The largest facilities currently have an installed capacity of over 1,000 MW, and power plants with a capacity of over 3,000 MW will be built in the near future.
Over the past few years, production costs and market prices for solar cells and ancillary equipment have been steadily declining, reducing their share of the total cost of building solar PV power plants. In this context, the role of electronic components, which do not directly depend on the development of solar technologies, significantly increases.
Large photovoltaic systems are usually equipped with a communications infrastructure that allows the operator to monitor and control energy production in real time.
This infrastructure can be used to detect damage to individual photocells and simplify maintenance.
In some regions, utility operators and even government agencies require appropriate communication interfaces for large solar power plants to ensure their compatibility with the grid.
The complexity of the communication equipment of a solar power plant increases many times over if the photovoltaic panels are equipped with tracking systems.
Biaxial tracking systems, which are commonly used in concentration photovoltaics (CPV), require decentralized control devices for each individual tracking system.
These units require communication interfaces to receive control and calibration commands and send information about their current status. In the largest power plants, the number of tracking systems can exceed 1000, which makes specific requirements for communication equipment.
Until now, engineering companies and electrical equipment manufacturers have concentrated their efforts mainly on improving solar cells and modules, since these are the most problematic and financially burdensome parts of any solar project. This has led to a technical lag in communication equipment for PV systems.
Not surprisingly, investors are now looking for new automated management, monitoring and maintenance solutions.
Solar power management systems can significantly reduce operating costs. For example, remote control replaces costly field service in remote areas. Modern solar PV power plants successfully use industrial Ethernet to communicate between key network elements.
When looking for a new solution, engineers are faced with two conflicting design criteria. On the one hand, this is a cost reduction due to the use of a simpler system. On the other hand, this is a reduction in maintenance and expansion costs due to a smart and scalable design.
Improving the efficiency of electricity production
Improving the efficiency of solar power plants starts with ensuring the proper operation of the communication system itself.The ability to self-configure and establish reliable connections between network elements are important factors.
The normal operation of tracking systems requires accurate observation of the sun. Consequently, distributed control systems need precise timing in order to be able to calculate the position of the sun. Other important information comes through wind sensors that protect sensitive equipment from damage.
The tracking systems of solar power plants are rapidly improving. In order to improve the accuracy of tracking the sun and thus energy production, the new trackers compare the level of energy production with neighboring PV modules in real time.
Therefore, a reliable communication system must be installed for maximum performance. In the case of photovoltaic tracking systems, this requires communication between the control center and the individual tracking systems.
Special attention should be paid to the situation when the solar power plant is connected to an energy storage system (for example, LAES) or other sources of electricity.
Monitoring systems for solar power plants
The operation of photovoltaic systems requires constant monitoring.Since most solar power plants are located in remote areas, operators must use all possible tools to keep up to date information on equipment performance and health.
Typically, O&M service providers have limited information on the impact of weather and landscape conditions on solar power plants. But in the event of a drop in performance, it is not always possible to quickly send a technical team and determine which component has a problem and whether the malfunctions are related to the weather.
Because of this, remote monitoring systems are the key to smooth operation. Remote monitoring can be carried out from the customer's office and from the office of the company responsible for the maintenance and repair of the PV system.
Regardless of the size of the power plant, comprehensive monitoring is essential for the minute-to-minute monitoring of energy production.
Solar systems are not only clean energy technology, but also a source of long-term income for investors.
For the fastest return on investment, equipment must run smoothly.
A qualitative analysis of the state of the system requires the collection of data from the local meteorological station, as well as information on the condition of photovoltaic cells, inverters, transformers and other equipment. Currently, solar photovoltaic power plants are monitored by electronic data acquisition, monitoring and control (SCADA) systems. These systems are used for various purposes and may differ significantly in their technical characteristics.
Monitoring is necessary for operators to identify emergencies at a solar power plant. This allows them to monitor critical parameters and prevent equipment failures. But the potential benefits of monitoring systems are highly dependent on the amount and set of recorded data.
Performance is just one of the many available variables that need to be monitored.
Reducing the cost of maintenance and repair
Optimizing the maintenance of solar PV power plants using electronic systems is an important factor in the success of an investment project.A well-designed monitoring and analysis system contributes to an increase in annual electricity production, a significant reduction in operating, repair and maintenance costs.
Regular monitoring of the amount of energy generated provides valuable information in cases where performance has decreased or there is a risk of equipment failure. Based on this data, preventive maintenance can be ordered to optimize system performance.
The cost of maintaining solar power plants is growing steadily every year due to aging equipment.
At the same time, monitoring and maintenance are becoming more and more important. Ensuring high levels of availability and productivity, reducing downtime and quickly detecting faults are of the highest priority.
Maintenance and expansion of equipment without expensive on-site setup saves a lot of money. This is made possible by systems for remote reconfiguration. Reconfiguration includes both changing certain parameters and firmware of devices.
Such systems allow a number of preventive procedures to be performed to ensure normal operation with maximum energy production without the need for engineers to visit the site. For example, cleaning of tracking systems can be done by robots using a wireless communication system for localization.
Operators can perform special tests to detect errors in communications, inverters, or photovoltaic modules.
Overvoltage protection of solar power plants
With several gigawatts of additional installed capacity each year, solar power plants are becoming an important part of the energy system in a number of countries.Today, large-scale photovoltaic systems with hundreds of megawatts are being installed and connected directly to medium and high voltage networks.
As part of the energy system, solar power plants must ensure the stability of its operation. It is important to reduce waste and minimize downtime.
With investment costs in the hundreds of thousands of euros per megawatt, solar projects require a careful analysis of the risk associated with overvoltage from a lightning strike. The results of these studies should be taken into account by engineers during the design stage of the photovoltaic system.
The importance of lightning protection
There is a clear statistical relationship between the level of solar radiation, humidity and lightning frequency.Studies show that regions of the world with high levels of solar radiation and humidity are most susceptible to lightning.
The frequency of lightning in the region (number of lightning strikes per square kilometer per year) and the location and size of the solar PV power plant are the basis for calculating the risk.
Problems in photovoltaic systems can occur as a result of direct lightning strikes and as a result of processes caused by an electromagnetic field. In addition, voltage peaks due to switching processes in AC circuits can damage photovoltaic modules, inverters, controllers, monitoring and communication systems.
These failures result in additional costs to replace or repair components, loss of performance and the use of redundant equipment. Lightning strikes can damage bypass diodes, power semiconductors and entire communication systems, which also increases maintenance and repair costs.
In some countries, grid operators have strict requirements for equipment availability.
Banks and insurance companies also usually require implementation of lightning protection measures for funded energy projects.
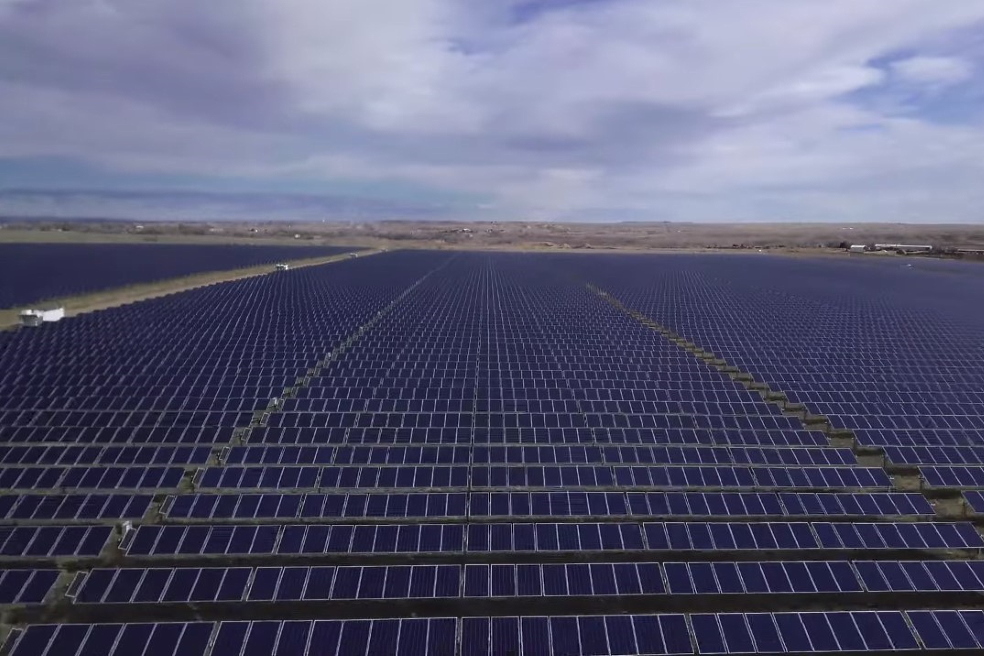
Construction of solar PV power plants: economic feasibility and cost
Any rise in oil prices on international markets draws the attention of investors, politicians and researchers to renewable energy sources.Solar energy has been controversial in past decades, but today it is clear that solar power plants will soon replace traditional energy sources.
The question is when will this happen.
Is it possible to state unequivocally that solar power plants are technically and economically more efficient than thermal power plants or nuclear power plants?
The widespread use of the technology depends on its current cost-effectiveness. Solar energy today cannot always compete with traditional sources. But the long-term trend is that fossil fuels will rise in price, and solar cells and ancillary electrical equipment will become cheaper.
According to BloombergNEF, in 2020 the cost of renewable energy has dropped significantly around the world.
The levelized cost of energy (LCOE) for large-scale photovoltaic systems has decreased by 4% compared to the second half of 2019 - to € 46 per MWh. Meanwhile, the base LCOE for batteries fell to € 138 / MWh, having fallen in price by 2 times over the past year.
In China, the largest solar market, solar LCOE has dropped to € 35 / MWh thanks to the introduction of more efficient PV cells. This is almost 9% less than in the second half of 2019. The cost of operating new solar power plants in the country is now almost equal to the cost of operating coal-fired power plants, at around € 32 / MWh.
According to BNEF reports, the cheapest photovoltaic projects financed in the first six months of 2020 should reach LCOE in the range of 21-27 € / MWh.
These projects in Australia, China, Chile and the United States will compete with the remaining fossil fuel power plants.
Meanwhile, Abu Dhabi-based EWEC has unveiled the results of the latest solar energy tender in the UAE for a 2 GW solar photovoltaic project. The operator plans to supply energy at a price of only € 12.46 / Mwh.
Solar power plants have a number of advantages over coal-fired TPPs and nuclear power plants:
• The construction of a solar power plant is much faster as the photovoltaic modules are easy to install and connect.
• It is easier for engineering companies to choose the location of the solar power plant in accordance with the infrastructure and terrain features.
• The construction of solar power plants in remote areas reduces the energy losses associated with long-distance transmission.
• Unlike traditional power plants, modular solar energy production can be smoothly expanded as consumption increases.
Solar power plants do not pollute air and water, maintaining an ecological balance. For this reason, solar energy production is stimulated by government initiatives in most developed countries.
Today, there is no longer any doubt about the economic feasibility of building solar power plants.
The time will come when solar energy will completely displace coal and gas from the energy sector.
Construction of solar power plants for industrial enterprises
Industrial enterprises are among the largest consumers of electricity in the world energy market.The huge number of equipment and machines requiring power, as well as the accompanying office premises of industrial companies determine the need for an uninterrupted and reliable power supply.
With the awareness of the negative impact of traditional energy sources on the environment, more and more enterprises are switching to renewable energy sources. The challenge is to reduce the carbon footprint at every stage of the manufacturing process.
Many companies, especially in the automotive and electronics sectors, are choosing solar power plants as the optimal technology for generating clean energy.
Industrial facilities use large amounts of energy in a wide variety of processes, which are usually designed for maximum intensity.
The annual electricity consumption for the average US enterprise, for example, is 95.1 kWh per 0.09 m², which is at least 10 times the annual consumption of a typical household. Most of the energy consumed in factories is used in the form of heat, with the remaining about 20% in the form of electricity.
According to the latest research, this ratio is changing. In the 1980s, factories used 25-50% less electricity than they do now. Experts predict that by 2030 the share of electricity in the energy consumption of industrial enterprises will reach 30%.
The electrification of industry not only opens up many opportunities, but also poses new challenges for business. An example is companies that make full use of the electricity generated by coal-fired power plants.
These producers contribute to the problem of greenhouse gas emissions, which, in turn, leads to increased energy costs due to additional environmental taxes. Renewable energy sources minimize these problems. Several studies have shown that the use of solar energy can lead to significant savings in energy costs for manufacturers.
As with many other technologies, the efficiency of solar power plants increases as the scale of construction increases. In 2019, the cost of building a solar PV system for small consumers was US $ 3 per watt. However, this figure drops to $ 1 per watt when it comes to systems with an installed capacity of more than 1 MW.
Compared to coal, solar power is a clear winner because modern technology makes it possible to produce energy cheaper every year.
Benefits of solar power plants for industry
One of the main goals of integrating a solar power plant into industrial processes is to provide sufficient energy during periods of peak demand, which usually coincide with the hours of maximum exposure to sunlight.This allows customers to switch to solar power on demand and limit their dependence on the grid, which offers opportunities for cost savings. Thanks to reliable energy storage systems, production processes at night can be partially supported by solar energy.
Another advantage of solar power plants for industrial facilities is their relatively easy maintenance.
Photovoltaic systems have no moving parts or complex mechanical components, so the likelihood of breakdown and equipment failure is much lower compared to wind turbines. In addition, solar cells do not generate noise, which increases their suitability both in terms of the environment and in terms of improving the working conditions of personnel.
Solar power plants provide high flexibility for business.
The use of electricity from the grid is often accompanied by restrictions imposed by the operating companies. By integrating PV systems into industrial production, companies can regulate their electricity supply and save on energy.
Industrial solar heating systems
As previously mentioned, most of the energy for industry is used in the form of heat.Although some industries, such as metallurgy, require very high heating temperatures, most operations require low or medium temperatures.
Low temperature heating is widely used in the textile industry, in the food industry for sterilization, in the mining industry for extracting various components, and so on.
Solar heating systems are suitable for generating heat at temperatures up to 150 ° C. These installations include solar collector networks in which a liquid (water, a mixture of water and glycol) is circulated. The heat exchanger transfers heat from the primary circuit to the process heating circuit in the form of hot water, air flow or steam, depending on the process requirements.
The system is capable of storing heat to compensate for changes in solar radiation as well as fluctuations in heat demand.
Experts point out the important environmental and economic benefits of solar thermal systems that industrial customers may be interested in.
The environmental benefits come from reducing harmful emissions, which depend on the amount of fossil fuels replaced directly or indirectly by renewable energy. Depending on the location, a solar heating system with a capacity of just 1.4 megawatts (2000 m²) save 175 tons of carbon dioxide.
Economic benefits are associated with energy savings and energy security of the business, including by limiting energy purchases. When it comes to energy costs and potential savings, there are three factors to consider that are important when comparing the prices of solar thermal energy.
These are the initial cost of construction, the lifespan and the actual efficiency of the system.
These factors depend on the location and type of equipment, so they vary significantly in different regions of the world. The average investment cost for industrial solar heating systems can vary greatly depending on the country, operating conditions and type of installation.
According to the International Energy Agency (IEA), investments in a large solar heating system in Europe range from $ 350 to $ 1,040 per kW.
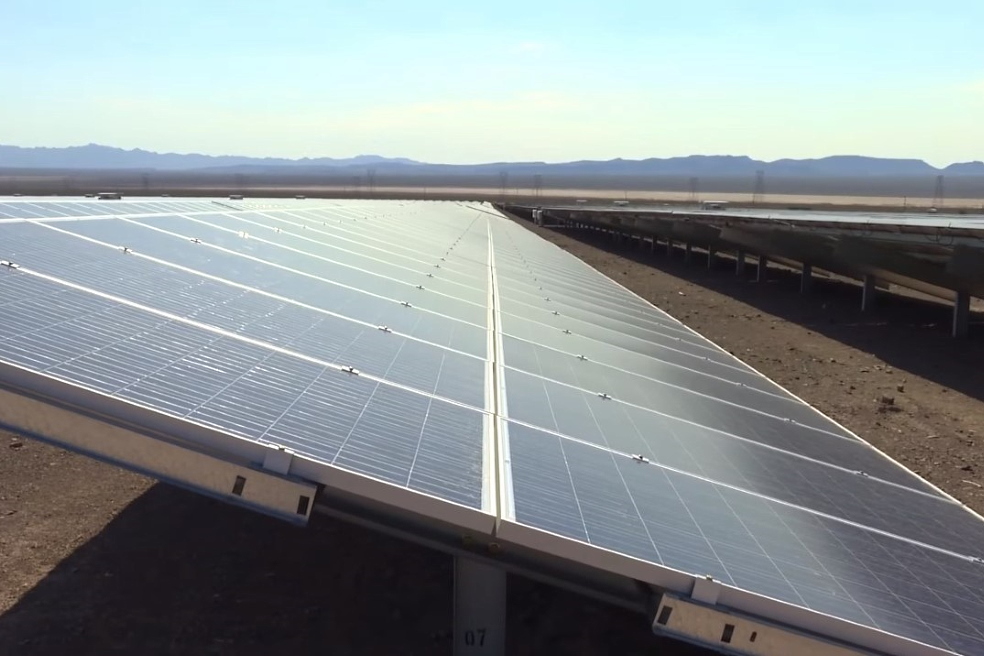
Important considerations for investors
Most industrial sites fully comply with all spatial requirements for the construction of a solar power plant.Typically, factories are built away from tall buildings or trees that can block sunlight.
Industrial buildings usually have a flat roof, which is ideal for installing PV modules. These roofs allow technical teams to install panels quickly and efficiently, which reduces construction time and cost. On such roofs, PV modules can be easily oriented towards the sun so that they can work with maximum efficiency.
For some industrial sites, ground-based solar systems are more suitable.
For example, when workshop roofs are rounded or not strong enough to support the weight of photovoltaic systems.
Most industrial sites have sufficient space for such systems.
The land around the facilities is usually occupied by parking lots and large fields separating them from the road network or residential areas.
These spaces are well suited for installing solar panels, which can be combined with panels and on rooftops, increasing the total amount of energy generated. An additional option is the solar sheds over the parking lots.
Currently, solar power plants require approximately 9 m² of free space for each kilowatt of installed capacity. Large industrial plants usually need several megawatts, so the area of solar panels must be well over 10,000 m².
Such spatial requirements are quite realistic for most factories. If roof area is not suitable or large plots of land are not available, an alternative solution is to install small solar panels in different locations.
Before proceeding with the construction of a solar power plant on an industrial site, it is necessary to consider several basic parameters.
Despite the availability of large building areas, it is advisable to analyze the energy needs of consumers. This will allow the future capacity of the solar power plant to be matched to the actual energy demand.
Energy-intensive businesses typically use a range of precision tools and equipment. Consideration must be given to the various types of equipment and their power ratings. This analysis should cover the energy consumption of all systems, including, for example, lighting and water filtration.
Every industrial plant has fixed shifts, and many factories operate in multi-shift mode. This requires adapting the power generation to the work schedule. Factories operating night shifts must store enough solar energy during the day to store excess energy in batteries.
Environmental benefits are often not a sufficient incentive for a producer to switch to renewable energy sources.
Investors need substantial economic benefits.
Despite falling prices, building a solar power plant for industrial use is still very expensive. However, this should not be the determining factor when deciding in favor or against investing in such a project.
Given the minimal need for maintenance, once the equipment is purchased and installed, solar energy becomes virtually free. In more than 25 years of operation for a typical solar power plant, the only significant maintenance cost will be replacing the inverters.
Preferential Rate Power Purchase Agreements (PPAs) can be an attractive alternative for some industrial enterprises.
Also common are agreements between installers or utility companies with factories on the mutually beneficial use of available space to generate green energy.
Engineering companies build and pay for the construction of power plants on the territory of the plant, while retaining ownership of the equipment. Factories are allowed to buy the generated solar energy at a specified price, and the tariff is usually attractive to an industrial customer.
These and other schemes of work ultimately contribute to the construction of new industrial solar power plants around the world.
EPC contracting in solar energy
The solar photovoltaic plant is a symbol of environmental responsibility, high return on investment and safety.The design and construction of such facilities is quite complex and time-consuming.
Against the backdrop of the rapid development of solar power plants, an additional question arose.
What is the best way to design and build innovative energy facilities in order to achieve optimal results with minimal time and resources?
In the coming decades, EPC contracting will prevail in the field of solar energy.
EPC (design, procurement and construction) is a special form of contracting that allows you to most effectively manage all aspects of the project implementation process of a photovoltaic installation. It covers everything from obtaining permits to design, construction, testing and commissioning.
Thanks to EPC contracting, the construction of solar PV power stations has become much easier, especially for small investors who do not have the resources to control every detail of the project.
Large international engineering firms are now responsible for every aspect of design and construction. Professional teams of engineers, lawyers and marketers minimize all kinds of risks that may affect the project, including delays in the installation schedule, problems with the acquisition of materials, obtaining official permits and putting into operation.
A good EPC contractor in the solar energy industry must have agreements with major international manufacturers to ensure that the best contractual conditions are achieved, as well as to ensure an uninterrupted supply of components and materials.
Engineering company specialists must strictly comply with all technical and legal standards, as well as ensure financial stability and reliability of the project.
The competence of the EPC contractor will include market research, design, logistics, installation, maintenance and much more.
If you choose EPC-contracting, it is extremely important to find a reliable partner who has a wide range of competencies and provides the necessary tools for implementing energy projects.
Such a partner will be a single point of contact for the customer.
If you need to design or build a photovoltaic plant, please contact us at any time convenient for you.
Our experts will answer your questions.